Focus on Electrophysiology | Atrial Fibrillation Mapping: New Perspectives?
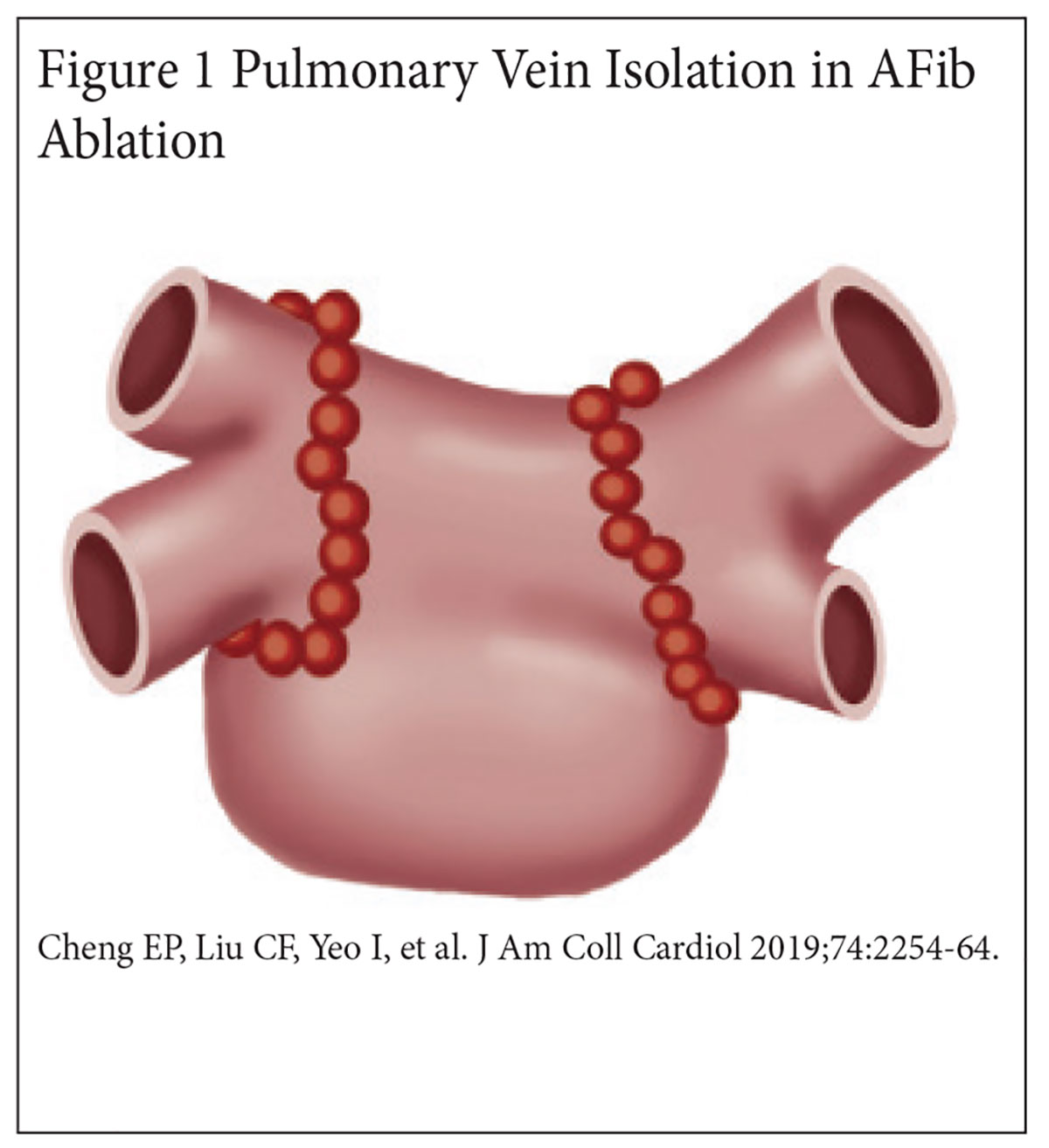
The turn of the 21st century was notable for several landmark studies that significantly advanced our management of atrial fibrillation (AFib).
In 1998, Haïssaguerre, et al., demonstrated that 94% of atrial ectopic foci initiating AFib were localized to the pulmonary veins (PVs), and focal ablation of these foci eliminated AFib in a majority of patients.1 In 2000, Pappone, et al., introduced the anatomical approach for PV isolation using circumferential lesion sets around PV ostia.2
In 2002, Marrouche, et al., became the first to describe what is now known as wide antral circumferential ablation for PV isolation3 (Figure 1).
Nearly 20 years later, these contributions have remained the cornerstone for current catheter-based ablation strategies in patients with paroxysmal AFib.4
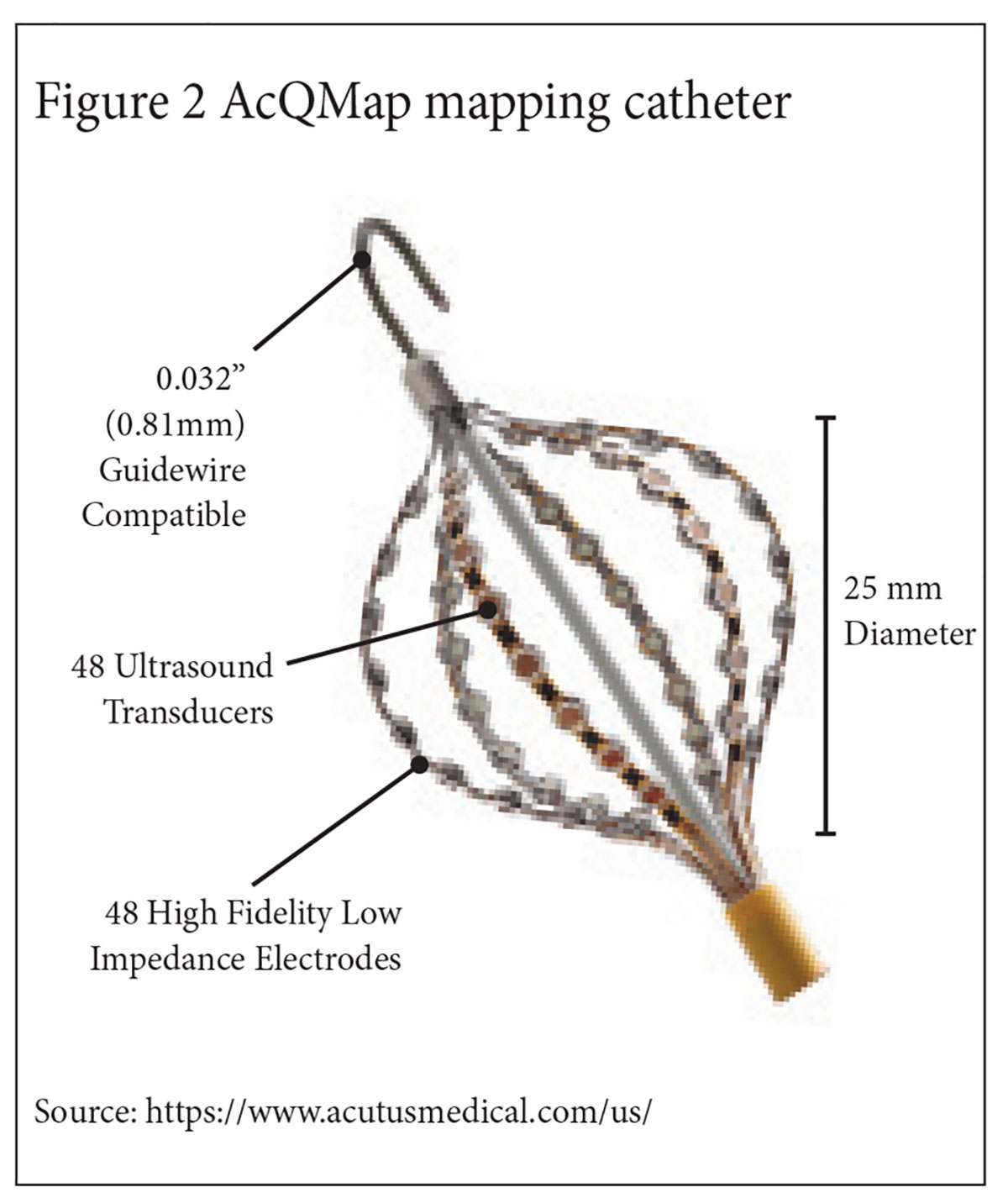
Unfortunately, PV isolation alone has been less effective in patients with persistent AFib. Additional ablation lesion sets targeting the posterior wall, complex fractionated electrograms, and various anatomical structures have led to mixed clinical results.
In 2015, the pivotal STAR-AF II trial revealed no significant difference in the rate of AFib recurrence at 18 months postprocedure between PV isolation alone, PV isolation with complex electrogram ablation and PV isolation with linear ablation (59% vs. 49% vs. 46%, p=0.15).5
As a result of these disappointing results, electrophysiologists have continued to search for the holy grail in persistent AFib ablation: non-pulmonary vein triggers.
Until recently, arrhythmia mapping technologies have primarily focused on elucidating the electrical properties of AFib, namely, the voltage amplitude of underlying cardiac substrate and the propagation pattern of the arrhythmia wavefront.
While this approach has been helpful in identifying areas of diseased tissue beyond the PVs, ablation of these abnormal areas has not substantively improved outcomes in patients with persistent AFib.6
Enter Acutus Medical, a newcomer to the arrhythmia mapping industry, that is taking a different approach to mapping AFib that examines the charge dipole density of the arrhythmia wavefront and the contractility of the underlying substrate.
By correlating the electrical and mechanical properties of atrial activity, this approach may ultimately discover the non-PV triggers that drive persistent AFib.
Charge Density Mapping
A voltage map of a cardiac chamber is typically performed using a roving catheter that comes into direct contact with the endocardial surface. The voltage measured is a combination of the local charge dipole source and numerous far-field sources.
If the component contributed by far-field sources at any given location becomes significant, it can lead to a voltage measurement that fails to accurately reflect local electrical activity.
Using a 48 electrode non-contact basket mapping catheter (Figure 2), the Acutus mapping system, AcQMap, is able to simultaneously measure endocardial voltage generated by a broad region of a cardiac chamber.
An inverse algorithm is then applied to the globally acquired voltage measurements to solve for the local charge dipoles at any given location within the cardiac chamber, reducing the signal interference from far-field sources.
The distribution and amplitudes of the local dipoles are then displayed by AcQMap as a charge density map. Similar to the voltage map, areas of abnormal charge density can be highlighted using a color spectrum.
In an initial validation study of the charge density map in a computer-simulated model of atrial flutter, the charge density map demonstrated a four-fold improvement in local signal resolution compared to a voltage density map.7
In a subsequent clinical validation of the charge density map in 20 patients with AFib, the AcQMap non-contact voltage map demonstrated comparable atrial signal measurements compared to a contact voltage map (with a cross correlation coefficient of 0.83 when the Acutus basket mapping catheter was ≤40 mm of the endocardial surface).8
In the recent UNCOVER AF trial, the use of AcQMap in 127 patients with persistent AFib to guide a non-pulmonary vein ablation strategy resulted in one-year arrhythmia-free outcomes of 73% after a single procedure and 93% after two procedures (Figure 3).9
Left Atrial Mechanical Function
From a research perspective, the most exciting feature of AcQMap is its ability to track cardiac chamber contractility. The Acutus non-contact basket catheter contains 48 ultrasound transducers aligned in parallel with its mapping electrodes.
Each of the ultrasound transducers is able to produce an M-mode tracing of the local endocardial wall within its line of sight. Over a cardiac cycle or predefined time frame, the regional displacement of endocardial surface can be determined.
By aggregating each regional contractility pattern, the global mechanical function of a cardiac chamber can then be derived (Figure 4).
The impact of left atrial (LA) contractility during AFib has been noted since the 1999 SPAF-III study, in which Goldman, et al., demonstrated that a left atrial appendage (LAA) emptying velocity of ≤20 cm/s was significantly associated with the formation of LAA thrombus.10
Since then, numerous studies have evaluated the periprocedural impact of LA mechanical function on ablation strategy, but none have investigated the intraprocedural impact of mechanical function during ablation.
There are many clinically relevant questions that can be answered by simultaneously analyzing the electrical and mechanical properties of atrial tissue.
What is the clinical relevance of cardiac tissue that contracts differently in sinus rhythm than in AFib? Is there a mechanical signature for diseased cardiac tissue that could make it a more favorable or less favorable ablation target?
Borrowing from concepts in interventional cardiology, can additional mechanical characterization of diseased electrical substate identify "viable" myocardial regions whose contractility could be salvaged with further ablation, or, similar to the impact of functional flow reserve, identify myocardial regions that are not clinically significant to warrant further ablation?
While it is known that ablation creates scar tissue and scar tissue can be arrhythmogenic, it is not known whether ablation, whether empiric or targeted, has any deleterious impact on LA mechanical function.
Is it possible to ablate "too much," such that the global mechanical function of the LA begins to deteriorate if the scar burden exceeds a certain threshold? Could postprocedural phenomena such as stiff LA syndrome be predicted by real-time, intraprocedural imaging of LA mechanical function?
Like all electroanatomical mapping systems, the AcQMap system has its share of inconveniences and limitations. The mapping system requires a 16 French venous ostomy to accommodate its steerable sheath (for the basket catheter) and a 10.8 French venous ostomy to accommodate its introducer sheath (for an electrical reference), which may be larger venous access points than what is currently used for analogous instruments.
While the ability to rapidly generate a non-contact LA with the basket mapping catheter is convenient, it may not accurately capture the geometry of PV ostia, which are often beyond the line of sight of the ultrasound transducers, or electrical signals beyond 40 mm from the catheter electrodes.
In some cases, a traditional contact mapping catheter may be required to clarify geometry or signals. Charge density mapping has not been validated in the literature to the same extent as voltage mapping, and the range of abnormal charge density measurements correlating with known values for low voltage and scar tissue remains unclear.
However, like all electroanatomical mapping systems, the AcQMap system will improve over time.
No mapping system is perfect, but few have demonstrated the potential to advance our understanding of AFib to the same extent as the AcQMap system, in terms of understanding the pathophysiology of complex arrhythmias not only from an electrical but also from a mechanical standpoint.
Only time will tell whether the novel metrics of charge density and left atrial contractility will significantly affect the future ablation strategy for persistent AFib, but for now the technology sure seems promising.
References
- Haïssaguerre M, Jaïs P, Shah DC, et al. Spontaneous initiation of atrial fibrillation by ectopic beats originating in the pulmonary veins. N Engl J Med 1998;339:659-66.
- Pappone C, Rosanio S, Oreto G, et al. Circumferential radiofrequency ablation of pulmonary vein ostia: A new anatomic approach for curing atrial fibrillation. Circulation 2000;21;102:2619-28.
- Marrouche NF, Dresing T, Cole C, et al. Circular mapping and ablation of the pulmonary vein for treatment of atrial fibrillation: impact of different catheter technologies. J Am Coll Cardiol 2002;40:464-74.
- Rottner L, Bellmann B, Lin T, et al. Catheter ablation of atrial fibrillation: State of the art and future perspectives. Cardiol Ther 2020;9:45-58.
- Verma A, Jiang CY, Betts TR, et al; STAR AF II Investigators. Approaches to catheter ablation for persistent atrial fibrillation. N Engl J Med 2015;372:1812-22.
- Clarnette JA, Brooks AG, Mahajan R, et al. Outcomes of persistent and long-standing persistent atrial fibrillation ablation: a systematic review and meta-analysis. Europace 2018;20(FI_3):f366-f376.
- Goldman ME, Pearce LA, Hart RG, et al. Pathophysiologic correlates of thromboembolism in nonvalvular atrial fibrillation: I. Reduced flow velocity in the left atrial appendage (The Stroke Prevention in Atrial Fibrillation [SPAF-III] study). J Am Soc Echocardiogr 1999;12:1080-7.
- Grace A, Willems S, Meyer C, et al. High-resolution noncontact charge-density mapping of endocardial activation. JCI Insight 2019;4:e126422.
- Shi R, Parikh P, Chen Z, et al. Validation of dipole density mapping during atrial fibrillation and sinus rhythm in human left atrium. JACC Clin Electrophysiol 2020;6:171-181.
- Willems S, Verma A, Betts TR, et al. Targeting nonpulmonary vein sources in persistent atrial fibrillation identified by noncontact charge density mapping: UNCOVER AF Trial. Circ Arrhythm Electrophysiol 2019;12:e007233.
Clinical Topics: Arrhythmias and Clinical EP, Implantable Devices, SCD/Ventricular Arrhythmias, Atrial Fibrillation/Supraventricular Arrhythmias
Keywords: ACC Publications, Cardiology Magazine, Pulmonary Veins, Atrial Fibrillation, Catheter Ablation, Atrial Premature Complexes, Catheters, Atrial Flutter, Heart Atria, Endocardium, Algorithms, Computers, Electrodes, Atrial Appendage, Cicatrix, Thrombosis, Atrial Function, Left, Transducers, Benchmarking, Technology, Ostomy, Electrophysiology, Electrophysiology
< Back to Listings